This page introduces you to the natural cycling of three elements that are critical for life—C, P, and N—and discusses how that cycling has been altered by human activity. The consequences of these alterations range from algal blooms to climate change.
Natural and Anthropogenic Cycles of Carbon, Phosphorus, and Nitrogen
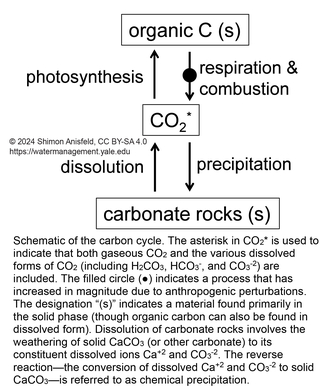
This figure is a simplified schematic of the carbon cycle, showing the processes of photosynthesis and respiration (discussed in more detail here), as well as the cycling of carbon between CO2 and carbonate rocks (mostly limestone, CaCO3). The two primary anthropogenic perturbations to this cycle bothinvolve the accelerated conversion of organic carbon to CO2, which is a greenhouse gas and the primary driver of climate change:
- Combustion of fossil fuels (coal, oil, natural gas) takes organic carbon deposits that have accumulated over millions of years and converts them to CO2. Combustion is similar to respiration in that it involves the exothermic oxidation of organic matter using O2.
- Land-use change—primarily deforestation—takes organic carbon in the form of vegetation (and soil organic matter) and converts it to CO2, either by burning it or by cutting it down and letting it decompose.
A third, smaller change to the carbon cycle is the conversion of CaCO3 to CaO and CO2 during the production of cement (not shown in this figure).
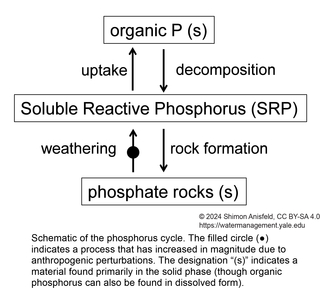
This figure is a simplified schematic of the P cycle. “Uptake” refers to the creation of cellular organic P compounds (such as nucleic acids) during photosynthesis. The pool of P that is available for uptake consists mostly of the simplest dissolved inorganic form of P (ortho-phosphate, PO43-) and was traditionally called Dissolved Inorganic Phosphorus (DIP). However, this pool is now referred to as SRP (Soluble Reactive Phosphorus) to acknowledge that some small organic P-containing molecules can also serve as P sources for algae, while some large inorganic forms, such as polyphosphates, can’t be used. “Decomposition” (also called mineralization) is the breakdown of large organic P compounds back to PO43- during respiration.
P can also be found in minerals such as hydroxyapatite, Ca5(PO4)3(OH); rock weathering releases P from these forms into biologically available forms, while formation of new rocks through geological processes returns P back into rock-bound unavailable forms. The release of P from rocks is much slower than its biological uptake, so the rate of P mineral weathering sets the amount of P available for biological productivity. The primary anthropogenic perturbation to the P cycle has been the mining of P minerals and their conversion to phosphate, mostly for use as agricultural fertilizer.
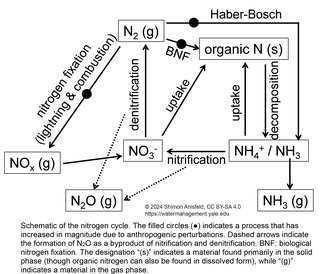
The N cycle is the most complex of the three. N has two primary dissolved inorganic forms—NH4+ (ammonium) and NO3- (nitrate)—referred to collectively as Dissolved Inorganic Nitrogen (DIN). Both forms of DIN can be used as nitrogen sources for the biological production of organic nitrogen compounds, such as proteins. Decomposition of organic nitrogen releases NH4+, which, in the presence of O2, can be converted to NO3- by microbes. Depending on pH, NH4+ can also be converted to NH3 (ammonia), which can volatilize as a gas. Denitrification—which occurs only in reducing environments—converts NO3- to N2, which is the most abundant form of N, but is very stable and not generally available for biological cycling. Both nitrification and denitrification lead to formation of the gas N2O as a byproduct.
We will use the term reactive N to refer to all forms of N except for N2, in order to distinguish between the actively-cycling, biologically-relevant forms, on the one hand, and the inert gas on the other. There are several ways that N2 can be converted to reactive N. Some specialized types of bacteria can use N2 as a nitrogen source, a process referred to as biological nitrogen fixation (BNF). N2 can also undergo fixation by reaction with O2 to yield NO and NO2 (referred to collectively as NOx) during combustion or through lighting strikes. Humanity has accelerated the creation of reactive N through three processes:
- Legumes have symbiotic relationships with N-fixing bacteria, so planting large areas of legumes increases reactive N in soils.
- Combustion of fossil fuels increases the rate of NOx production.
- An energy-intensive industrial process known as the Haber-Bosch process converts N2 to NH3, for use as an agricultural fertilizer and in industrial applications.
Thanks to the Haber-Bosch process, there is no risk of humanity running out of N (unlike P), but the environmental impacts of the acceleration of the N cycle are far-reaching.
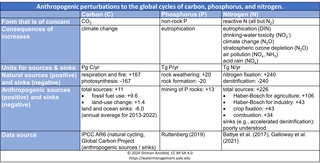
This table shows the magnitude of natural and anthropogenic fluxes of these three elements. Before anthropogenic disturbance, the natural carbon cycle was approximately balanced, with about 170 Pg of C undergoing photosynthesis each year, and a similar amount undergoing respiration. Anthropogenic impacts (mostly fossil fuel combustion and land-use change) are currently adding an additional 11 PgC/yr of CO2 to the atmosphere, of which about half is being removed from the atmosphere though natural processes on land and in the oceans; these land and ocean carbon sinks reflect the fact that higher atmospheric CO2 drives higher photosynthesis and air-water transfer. The portion remaining in the atmosphere is the main driver of climate change, while the portion in the ocean causes ocean acidification.
The mining of P rocks has led to a 65% increase in the rate at which P is made available for biological uptake, fueling growing concerns over both the environmental impacts of excess P (primarily eutrophication) and the potential depletion of the world’s supply of accessible P-containing rocks.
The N cycle has experienced a larger anthropogenic impact than either C or P, with humanity’s annual production of reactive N now about equal in magnitude to natural production. The extent to which natural N sinks—particularly denitrification—have increased in response to higher N availability is poorly understood, but we do know that our acceleration of the N cycle is driving a variety of impacts, sometimes referred to as the “N cascade”:
- DIN drives eutrophication of coastal and marine water bodies.
- NO3- (nitrate) is toxic to people when present at high concentrations in drinking water.
- N2O (nitrous oxide) is a greenhouse gas and a contributor to the depletion of stratospheric ozone.
- NOx is an air pollutant that contributes to the formation of photochemical smog. It can also be oxidized in the atmosphere to form nitrate aerosols (a component of unhealthy particulate matter) and nitric acid (a component of acid rain).
- NH3 (ammonia) in the atmosphere is a harmful air pollutant and can contribute to the formation of nitrate and sulfate aerosols.
- Atmospheric deposition of N (originating as either NOx or NH3) can bring DIN—and thus eutrophication problems—to remote areas.