This page explores the remarkable chemical and physical properties of the molecule H2O, and the ways in which those properties affect its environmental behavior.
Water as a Molecule
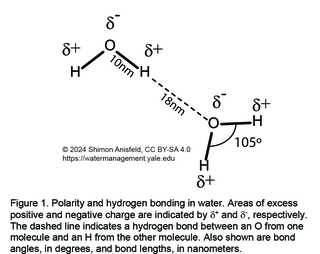
Water is a polar molecule. What this means is that electronic charge is not evenly distributed among the 3 atoms of the water molecule (Figure 1). In particular, the O atom—which is more electronegative than the H atoms (i.e., it exerts a stronger pull on electrons)—takes more electrons than its share, gaining a partial negative charge, while the H atoms become electron-deficient, i.e., positively charged.
When two water molecules are near each other—as is the case in the liquid and solid states of water, but not the gas state—the proximity of a negative O from one molecule to a positive H from a second molecule leads to a special kind of inter-molecular attraction, called a hydrogen bond (Figure 1). Since each water molecule can form up to 4 hydrogen bonds, a drop of water can be thought of as a dynamic network of millions of hydrogen bonds that hold together the individual water molecules rather tightly.
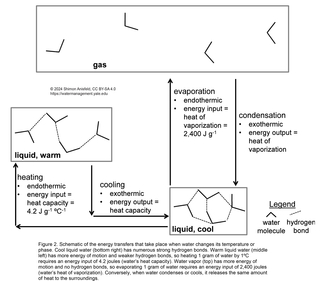
One consequence of water’s hydrogen bonding is that significant inputs of energy are required to pull water molecules apart from each other, since doing so will weaken or break the hydrogen bonds. There are two common situations in which water molecules are pulled away from each other (Figure 2): evaporation (since water molecules in the gas state are too far apart to interact with each other) and heating (since warmer water has molecules that are moving faster and are, on average, farther apart). Thus, hydrogen bonding causes water to have a high heat of vaporization: the amount of energy required for evaporation; and a high heat capacity: the amount of energy required to increase the temperature by a given amount. Put another way, evaporation and heating of water are highly endothermic processes; they require large inputs of energy. Conversely, condensation and cooling of water are highly exothermic processes; they release large amounts of energy.
This ability of water to transfer energy through temperature and phase changes is responsible for the close linkages between the Earth’s energy and water cycles. For example, the evaporation of water, followed by condensation in clouds, is an important way that heat is transferred from the Earth’s surface to its atmosphere, thus regulating surface temperature. Conversely, the hydrologic cycle is driven by solar energy, and spatio-temporal patterns of precipitation are governed by the distribution of energy within the ocean-atmosphere system. This also means that “climate change is water change”; one of the most significant impacts of the increased heat in the Earth system will be changes in the magnitude and timing of local water availability.
A second consequence of water’s hydrogen bonding and polarity is its ability to dissolve other polar substances (a phenomenon sometimes described as “like dissolves like”). Thus, when you put table salt (NaCl) in your soup, it easily dissociates into its constituent ions Na+ and Cl-, since the polar environment that water provides can readily accommodate those charged atoms. Similarly, when you put sugar (the organic molecule sucrose, C12H22O11) in your coffee, it easily dissolves because it is itself a polar molecule. (Unlike the ionically-bonded NaCl, which breaks into Na+ and Cl- upon dissolving, the covalently-bonded sucrose remains intact when it dissolves.)
Water’s ability to dissolve ions is critical to rock weathering. Near the Earth’s surface, naturally acidic water slowly leaches the more soluble elements (especially K+, Na+, Mg2+, and Ca2+) from rocks, making them available to plants and ultimately releasing them to rivers and the ocean. Over geologic time, the ocean has accumulated these salts, to the point where every kg of seawater has about 35 g of dissolved salt (3.5% or 35 parts per thousand). Note that the ocean is no longer accumulating salt, but is instead roughly at steady state with respect to ions, meaning that the input of ions from rivers to the ocean is balanced (over relatively long time scales) by various losses, such as formation of evaporite minerals and reaction with underwater volcanos.
In contrast to ions and polar molecules, non-polar molecules—those that have relatively uniform charge distribution due to the absence of oxygen and other electronegative atoms—dissolve poorly in water and are termed hydrophobic. Hydrophobic substances include vegetable oils, animal fats, petroleum (and petroleum products such as gasoline), and a variety of synthetic chemicals. Rather than remain in water, these chemicals tend to accumulate in sediment and biota, or, when present at high levels, form a water-insoluble phase such as an oil slick. Still, water can be an effective medium for transporting even hydrophobic chemicals, since moving water can carry large amounts of suspended sediment and its associated contaminant load. In addition, even low aqueous concentrations of some of these chemicals can be concerning because of their high toxicity.
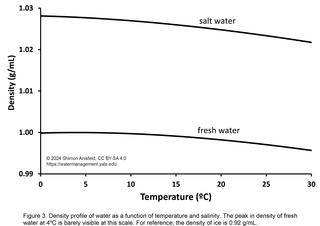
One final consequence of hydrogen bonding has to do with water’s density (mass per unit volume). Most substances are denser in the solid state than in the liquid state, but for water, the opposite is true; ice is less dense than liquid water because it forms a relatively open structure that optimizes its hydrogen bonds and takes up more space per unit of mass. In fact, as it melts and warms, fresh water increases in density up to 4ºC, after which it decreases in density again as the warmer, more rapidly-moving water molecules start to take up more space (Figure 3). Salt water has considerably higher density than fresh water, and continually decreases in density as it warms from its freezing point (Figure 3).
Because of water’s variable density, relatively deep water bodies such as lakes, reservoirs, estuaries, or oceans can undergo density stratification, in which lower-density near-surface water floats on top of denser, deeper water, creating two separate water masses that don’t readily mix. Density stratification is driven by some combination of salinity stratification (fresh water overlying salt water) and thermal stratification (e.g., 15ºC water overlying 5ºC water).